Biomechanical modeling of the central nervous system
Cerebrospinal fluid and Chiari type 1 malformation
The brain and spinal cord are surrounded by a clear, water-like liquid called the cerebrospinal fluid (CSF), which has an important protection and transport function in the central nervous system. Disruption of the normal production, absorption and/or dynamics of CSF plays a role in several neurological disorders such as hydrocephalus, Chiari malformation and even Alzheimer’s disease [1].
In Chiari type 1 malformation a local obstruction of the CSF flow is caused by the lower part of the cerebellum (tonsils), which herniates and extends below the foramen magnum, the opening in the skull base that enables brain-spinal cord connection, as shown in Figure 1. The obstruction is thought to be the cause of fluid accumulation inside the spinal cord (known as a syrinx), which is found in about 66% of patients with Chiari type 1 malformation. The syrinx may evoke severe motor and sensory symptoms by stressing the spinal neurons and eventually results in irreversible neural damage [2]. Currently, the only treatment is an invasive surgical procedure, which aims to remove the disturbance of the CSF flow. Because the procedure is invasive with a high risk for complications and variable outcomes, the interest raises to explore new treatments [3].
Although the principal manifestation of the disease is of a neurological nature, it is thought that biomechanical factors are at its origin. Thereby, the obstruction of the CSF flow leads to abnormal CSF pressures with implications on the mechanics and deformations of the soft tissues of the brain and spinal cord. Therefore, we aim to gain more insight into the CSF dynamics and the mechanical behavior of the neural soft tissues. With biomechanical models, both experimental and computational, we are able to investigate this in detail without the need to perform invasive measurements.
Figure 1: T2 MRI image of a patient with Chiari type 1 malformation showing the herniation of below the foramen magnum (red line) of the lower part of the cerebellum (1), the fluid-filled cavity (2), and the brainstem (3).
Computational models
The biomechanical behavior of the CSF and the neural soft tissues is investigated using different numerical tools in order to predict this mechanical behavior in detail. The applied techniques include computational fluid dynamics for the fluids and the Finite element modeling to study soft tissue behavior.
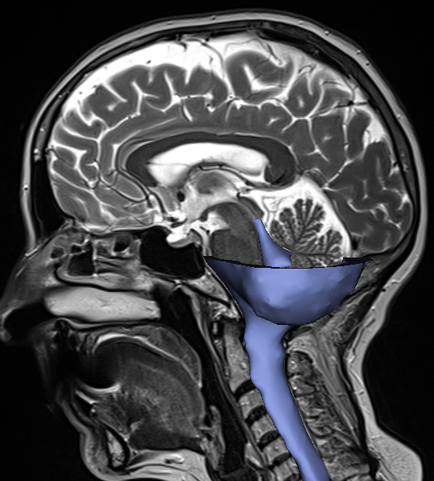
Computational fluid dynamics
Computational fluid dynamics (CFD) is a numerical technique that allows us to calculate the flow within a predefined volume. For the CSF, this three-dimensional volume is extracted from magnetic resonance images (MRI) of the head, as shown in Figure 2. The natural flow of CSF is affected by several internal and external factors with an important role of other cerebral fluid systems including the cardiovascular and the lymphatic system. For instance, biological mechanisms such as CSF production and absorption and pulsations of the arteries supplying the brain change the fluid dynamics. Hence, to capture the flow in a realistic way, it is necessary to incorporate those mechanisms. The resulting CFD models allow us to investigate in detail what happens in a healthy person and what goes wrong or changes in persons with neurological abnormalities.
Numerical software: Fluent (Ansys Inc.)
Finite element modeling
The structural behavior of soft tissues is determined by the loads and/or displacements applied at the boundaries and the material characteristics of these tissues. We quantify the deformations and stresses of the neural soft tissues as part of the structural behavior under different conditions using Finite element (FE) modeling. The brain and spinal cord are complex soft tissues because of their heterogeneous constitution of neurons, connective tissue and glial cells and their compliant (highly deformable) nature. Therefore, realistic modeling of the brain response to mechanical loading is challenging and requires a trade-off between complexity and feasibility.
Numerical software: Abaqus (Dassault Systèmes Inc.)
Experimental models
Next to computational models, we also study the behavior of the fluids and soft tissues in the central nervous system with experimental set-ups. To study the CSF flow, the effects of various boundary conditions on the flow of CSF is assessed by performing continuous measurements of pressure and flow. Thereby, the deformations of the brain tissues are experimentally measured under different loadings. The insights from these experimental models are compared to those obtained in the numerical models.
References
- Martin, B. A., & Heidari Pahlavian, S. (2019). Chapter 5 - Anatomy and Physiology of Cerebrospinal Fluid Dynamics (R. R. Lonser, M. Sarntinoranont, & K. B. T.-N. S. D. D. Bankiewicz, eds.). https://doi.org/https://doi.org/10.1016/B978-0-12-813997-4.00005-0
- E. C. Clarke, D. F. Fletcher, M. A. Stoodley, and L. E. Bilston, “Computational fluid dynamics modelling of cerebrospinal fluid pressure in Chiari malformation and syringomyelia,” J. Biomech., vol. 46, no. 11, pp. 1801–1809, Jul. 2013.
- S. Chotai and A. Medhkour, “Surgical outcomes after posterior fossa decompression with and without duroplasty in Chiari malformation-I,” Clin. Neurol. Neurosurg., vol. 125, pp. 182–188, 2014.